Investigation on Multifractal Dynamics of Human Heartbeat byPhysiological
Cardiovascular Model
Multifractality of healthy human heartbeat has attracted interest, because autonomic pathologies with certain diseases are known to be associated with altered heart rate multifractality. The results of recent studies on the heart rate multifractality include : Heart rate of healthy subjects who is administered beta-blocker has multifractality with the Hurst exponent of 0.1, while that of subjects who suffer from congestive heart failure (CHF) or is administered vagal blocker has monofractality with the Hurst exponent of 0.2. Further, heart rate of patients who suffer from primary autonomic failure (PAF) has multifractality associate with the Hurst exponent of 0.23.
To investigate intrinsic as well as complex cardiovascular mechanisms responsible for the mutifractality and its loss in, e.g. diseased conditions, further experimental investigations in humans might shed light. But human physiological experiments are associated with problems such as the limited length of data and intrinsic nonstationarity. Instead, here we develop a physiologically plausible structural model. We then conduct, by using this model, some “virtual” physiology experiments searching for the origin(s) of multifractal heart rate dynamics, and also show that the virtual disease would lead to changes in heart rate multifractality observed in the actual patients.
The model (Fig.1) is a system of delay-differential equations and a modification
of one initially proposed by Seidel and Herzel to study deterministic nonlinear
cardiovascular dynamics. It consists of i) neural afferents from baroreceptors
to the central nervous system, ii) autonomic (sympathetic and vagal) neural
efferents from the brain stem cardiovascular centers, iii) mechanical signal
transductions within the cardiovascular system finally setting the arterial
blood pressures, and iv) the effect of the baroreceptor afferents on the
instantaneous phase of respiratory oscillator. We set the balance between
sympathetic and parasympathetic activities influencing the phase velocity
of cardiac pacemaker so that responses in mean heart rate of the model
during selective or simultaneous blockades of the two branches of the autonomic
nervous system follow what are observed in actual physiological settings.
Then, the model was driven by two fractional Brownian motion added, at
each heartbeat, to the baroreceptor afferent activity (ξ1: global Hurst
exponent is 0.5) and the autonomic neural efferents (ξ2: global Hurst exponent
is 0.1).
In this study, the gains of i) vagal influence on the heart, ii) sympathetic influence on the heart, and iii) sympathetic influence on the vascular smooth muscle were tuned to simulate data with pharmacological blockades and/or autonomic pathologies.
A set of delay-differential equations was numerically integrated by a Runge-Kutta method of fourth order with a constant step size (5ms). In all simulations, we analyzed 20 sets of 50,000 heartbeat intervals as follows.
In this paper, the multifractal index and global Hurst exponent were calculated. For the multifractal index, τ(q) was calculated by wavelet transform modulus maxima method and its averaged curvature was used. The global Hurst exponent was calculated by detrended fluctuation analysis.
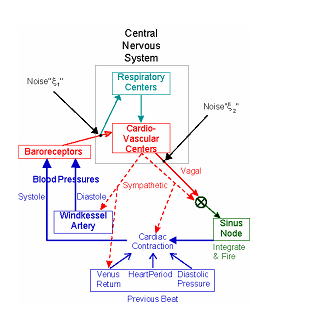
Fig.1 Schematic diagram of cardio-vascular model
The results for the multifractal indices and global Hurst exponent are shown in Fig.2. Negative values in averaged curvature indicate multifractality. It has been shown that the actual data with atropine and for CHF show smaller multifractality while those for PAF show almost comparable or even greater multifractality than the heart rate for healthy individuals. The results of statistical analysis for the simulated data generally have such tendencies. The model has almost the same level of multifractal index and global Hurst exponent as the actual healthy, pathological, and pharmacologically nerve-blocked data.
However, there are still some differences from the reality. For example, simulated data mimicking PAF show greater multifractality and smaller global Hurst exponent compared with the results for the actual data. The actual PAF data are characterized by the presence of abrupt changes (burst and/or step-like changes) that might lead to greater multifractality and larger global Hurst exponent. This seems to be caused by the loss of sympathetic influence on the vascular smooth muscles, resulting in instability of blood pressure and venous return responses. Although our model also has a mechanism with which the sympathetic influence on the vascular smooth muscles changes blood pressure and venous return, the effect is considered to be smaller compared to that in the actual PAF. This should be solved in the future research.
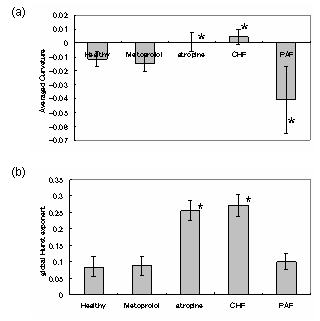
Fig2 (a): Averaged curvatures and (b): global Hurst exponent of the simulation. *indicates there is a statistical difference between the result of healthy condition (P<0.05).
|
|